Cardiogenic Shock: Epidemiology, Aetiology and Prognostic Considerations
The term cardiogenic shock (CS) refers to a series of complex and heterogeneous clinical scenarios characterised by primary myocardial dysfunction leading to the inability to maintain adequate tissue perfusion, resulting in progressive irreversible multi-organ failure.1
CS complicates up to 13% of acute coronary syndromes (ACS), which are traditionally considered the most prevalent cause.2 However, the increasing burden of chronic heart failure (HF) worldwide has changed the aetiological epidemiology in recent years. For example, recent data from the Critical Care Cardiology Trial Network underline the rising incidence of CS complicating acutely decompensated heart failure (ADHF), which represented up to half of that CS cohort.3 The timely identification of progression to overt CS in this group of patients may be more challenging. This is because of their chronically compensated low-output state and the more subtle manifestation of typical signs and symptoms.4 Moreover, even if significant heterogeneity has been described according to the aetiology and the severity at presentation, the prognosis of this acute condition remains very poor, with a short-term death rate of up to 50%.5–7
Notably, apart from the well-established benefit of early revascularisation of the culprit lesion in CS complicating ST-elevation MI, no further interventions to date have proven any survival benefit.8 In particular, the adoption of mechanical circulatory support (MCS) devices has been tested in randomised controlled trials (RCTs) among heterogeneous populations of CS patients without survival improvement.9,10
Many concerns about device-related complications have been highlighted. Nevertheless, many issues may negatively influence these results. These include the advanced impairment of included patients, the risk of pre-selection bias and difficulties in conducting large RCTs in such critical scenarios. Moreover, the complexity of the disease itself may deserve a comprehensive and prespecified management protocol.
Accordingly, some observational evidence suggests that the use of a ‘shock team’ approach using a pre-established therapeutic protocol is associated with significantly reduced short-term mortality.11–14 All these studies have strengthened the prognostic implications of early diagnosis, early adoption of MCS (when indicated) and continuous and dynamic re-evaluation of whether to upgrade, wean or move to a palliation strategy. Furthermore, the studies have largely adopted invasive haemodynamic monitoring with extensive use of the Swan-Ganz catheter to guide the different phases of the proposed protocols.
Historical Background of the Swan-Ganz Catheter
The Swan-Ganz catheter recently reached its fiftieth anniversary since the first use in a human body in 1969.15 Jeremy Swan firmly pursued its ideation and development – with the help of Willie Ganz and other colleagues – in the belief that bedside invasive haemodynamic monitoring of patients admitted for an acute MI (AMI) may assist in better therapy selection and, consequently, improved survival.
The pioneering study of Forrester et al. on bedside invasive haemodynamic monitoring in AMI patients demonstrated the effect of medical therapy according to the patient’s haemodynamic phenotype and led to the development of the well-known Forrester classification.16 This classification relates pulmonary capillary wedge pressure (PCWP) to cardiac index (CI) to categorise the patient according to their congestion and perfusion status with well-demonstrated prognostic and therapeutic implications.
In the decades that followed, the pulmonary artery catheter (PAC) became a hallmark of intensive care monitoring despite the lack of data on safety, accuracy and benefits of this technique. However, after an initial moratorium on PAC use in the mid 1980s, a propensity-matched analysis in the SUPPORT study in 1996 found higher mortality in a heterogeneous cohort of intensive care unit (ICU) patients receiving PAC.17,18 The same ominous prognosis was also described in the setting of AMI, namely the subject of the Forrester classification.19,20
In 2003, the first of several RCTs found no benefit of PAC on surgical patients.21 The publication of the ESCAPE trial in 2005 represented a cornerstone in the history of the Swan-Ganz catheter in HF: 433 patients with severe symptomatic decompensated HF, excluding those with CS, were randomised to receive therapy guided by clinical assessment alone or with the addition of PAC in order to target decongestion.22 Both groups experienced a reduction in symptoms and signs of congestion, although patients with PAC were more likely to receive vasodilator therapy and showed a faster time to resolution of symptoms. However, a neutral result was found in terms of the primary endpoint of days alive out of hospital in the first 6 months. Interestingly, patients screened but not randomised and receiving PAC monitoring were included in a separate registry, which reported a significantly higher mortality rate than those in the trial, underlining the clinicians’ common belief that invasive monitoring was necessary for more severe patients.23
Following these negative study results, many authors discouraged the routine use of PAC in the ICU and surgical settings.24 Many limitations of the tool were suggested to justify this, including the risk related to the invasive procedure itself (although inversely related to the centre’s experience), the challenge in obtaining accurate data, and – likely most importantly – the difficulty in correctly interpreting this information and responding with appropriate and standardised medical treatment, along with the advances in non-invasive diagnostic techniques.
Nonetheless, even if there was an initial progressive reduction in PAC use for the HF setting in general hospitals, it was followed by an increasing trend of usage, especially in large academic centres in the United States.25,26 One potential explanation for this paradox may be a shift in the clinical profile of patients admitted for HF towards more severe stages, leading clinicians to search for more accurate haemodynamic monitoring, in particular when considering advanced HF strategies.27
Limitations of Noninvasive or Minimally Invasive Techniques in the Cardiogenic Shock Setting
The use of echocardiography in the critical care setting – as opposed to the PAC – has burgeoned over the last decades. There are many potential benefits of this technique that may explain the trend. Firstly, echocardiography has a complementary role to invasive monitoring because it allows rapid evaluation of biventricular function and identification of severe valvular, pericardial and large-vessel disease or mechanical complications, helping to put in place adequate aetiological treatments. Moreover, thanks to its wide availability and easy handling, it has been largely proposed and used to non-invasively estimate haemodynamic data.28 It is well established that echo-derived haemodynamic estimators have prognostic implications even in the critical care setting.
Jentzer et al. recently published a large retrospective cohort of CS patients, showing how high E/e’ ratio and low echo-derived indexed stroke volume correlated with Society for Cardiovascular Angiography and Interventions (SCAI) stages and short-term mortality.29 However, the dynamic and rapidly changing nature of CS requires continuous monitoring techniques to provide reliable and fast estimation of haemodynamic parameters in each critical phase in order to promptly select the therapeutic strategy. Time-consuming averages of several echo-derived parameters are needed to get a raw range of value. Indeed, several practical problems may limit the suitability of echo-dynamics in the critical care setting and very few studies with small sample sizes have demonstrated reliability in this setting.30
Ultrasound windows may not always be permissive in clinical practice, especially in mechanically ventilated patients forced to the supine position. For example, inferior vena cava diameter and its respiratory variations, used to determine right atrial pressure (RAP), may not be obtained in up to 22% of cases – even in the hands of expert clinicians.31 Moreover, there may be suboptimal correlation with central venous pressure and many situations (e.g. right ventricular dysfunction or pulmonary hyperinflation) make this value unreliable for predicting fluid responsiveness.32,33
Further issues have to be acknowledged when estimating left filling pressures with echocardiography: the E/A ratio is dependent on diastolic function and it is not available in cases of AF; conflicting data have been published on the correlation between E/e’ and invasive PCWP, particularly in the setting of ADHF and if left bundle branch block or CRT stimulation is present; and the feasibility of pulmonary venous flow velocity evaluation is demonstrated only in an ambulatory setting, as are the majority of the correlation studies published on this theme since now.30,34–36 Finally, echo-derived cardiac output (CO) from the continuity equation has shown good correlation with the invasively-derived one in older observational cohorts.37,38 Notably, this technique is not reliable in cases of significant aortic regurgitation because of over estimation and is highly limited by inter-operator accuracy in the measurement of left-ventricular outflow tract diameter. Additionally, when a trans-aortic ventricular assistance device such as the Impella is used, it is further limited by significant beat-to-beat variability and device-related artefact.
Likewise, minimally invasive techniques have been proposed as alternatives to PAC monitoring. Those based on arterial waveform to estimate CO rely on external calibration (e.g. PiCCO [Pulsion], LiDCO [LiDCO], EV-1000 [Edwards Lifesciences]), internal calibration (e.g. Pulsioflex [Pulsion Medical Systems], LiDCO Rapid [LiDCO], FloTrac Vigileo [Edwards Lifesciences], Retia [Retia Medical]), or no calibration (MostCare Up PRAM [Vygon, Vytech]). Despite increasing interest in these devices in recent years following technological improvements, results in terms of CO estimation are controversial and further studies are still in progress (e.g. NCT04955184).39–41 Notably, the derived measures are significantly influenced by vascular impedance and are therefore less reliable in estimating haemodynamic data in the unstable CS setting: tachycardia, arrhythmias or MCS devices may interfere with the inferential models used to predict CO from pulsatory pressure curve, making these values less reproducible.42 In addition, these tools may require time-consuming multiple recalibrations, limiting their practical applicability in this context. Finally, transpulmonary thermodilution methods are limited by the inability to discriminate between right- and left-heart dysfunction or to accurately estimate left filling pressures.
Indications and Implications of the Swan-Ganz Catheter in Cardiogenic Shock
To date, the use of PAC monitoring in the CS setting is only recommended if there are uncertainties on diagnosis or for the most severe cases that are unresponsive to the first therapeutic attempts.43 Potential practical uses in CS were proposed in the SCAI/Heart Failure Society of America 2017 expert consensus document on invasive haemodynamics for the diagnosis and management of cardiovascular disease (Figure 1).44
The Role of the Pulmonary Artery Catheter in the Diagnosis and Classification of Cardiogenic Shock
The diagnosis of CS is usually made when the combination of clinical signs and symptoms of low CO and tissue hypoperfusion are matched in the presence of adequate intravascular volume.45 The most commonly used definition is the presence and persistence of systolic blood pressure (SBP) <90 mmHg, along with clinical or laboratory evidence (i.e. lactate elevation) of tissue hypoperfusion or the need for pharmacological or mechanical support to reverse it. However, it has been shown that the clinically estimated haemodynamic profile is comparable to the invasively derived one in the critical care setting in only half of cases, and non- invasive techniques have several limitations in this setting as previously mentioned.46 Therefore, invasive evaluation by right heart catheterisation (RHC) remains the gold standard for diagnosis.
A CI ≤2.2 l/min/m2 associated with a PCWP of at least 15 mmHg has been the traditional haemodynamic criterion for left-sided CS since the pioneering SHOCK trial in the AMI setting; furthermore, nowadays it is well known that CS is an evolving and multifaceted haemodynamic scenario.8 Invasive haemodynamic data from sub-studies of the SHOCK trial registry demonstrated that – beyond the criterion of reduced CI – the relationship between PCWP and systemic vascular resistance (SVR) can define different entities.47 The most frequent is the ‘wet and cold’ scenario in which both are elevated, accounting for up to two-thirds of AMI-related CS. ‘Dry and cold’ CS is characterised by hypoperfusion along with reduced filling pressure values or those within the upper range of normal. This represented up to 28% of post-MI CS in the SHOCK trial registry. Moreover, the loss of the compensatory increase in SVR caused by the cytokine storm resulting from systemic inflammatory response syndrome and/or ischaemic gut bacterial transmigration represents the dramatic scenario of ‘mixed vasodilatatory CS’.5
There has been growing interest in recent years in the spectrum of the pre-shock and non-hypotensive shock conditions characterised by normotension or relative hypotension with reactive tachycardia and initial – but often subtle – signs of end-organ hypoperfusion.44,48,49 For example, in the SHOCK trial registry it was demonstrated that up to 5% of patients had SBP >90 mmHg without any therapeutic support despite similar PCWP and CI values at RHC when compared to those of the classic CS haemodynamic phenotype. This was the consequence of higher compensatory vasoconstrictive tone as demonstrated by the higher SVR values.50 Considering the 43% rate of short-term mortality described in this subgroup of patients, under recognition or late recognition may have dramatic consequences.
Evaluation of Right Ventricle Dysfunction Using a Pulmonary Artery Catheter
The use of the PAC provides a unique opportunity to finely characterise the presence and the degree of right ventricle (RV) dysfunction alone or in combination with the left-sided dysfunction.
Up to 40% of AMI-CS patients show some degree of RV failure and up to 15% have severe dysfunction. A recent CS registry demonstrated that up to 16% of these patients had RV failure as the primary cause, with short-term survival similar to those with predominantly left-sided failure despite a better admission profile.51,52 Several haemodynamic predictors have been proposed to identify the failing RV. Among them, evidence of a pulmonary artery pulsatility index (PAPi) <0.9 has been described as the strongest indicator of severe RV failure and worse prognosis after MI.53
Guiding Supportive Pharmacological Therapeutic Interventions, Mechanical Circulatory Support Device Selection, Up-titration and Weaning
The accurate identification of the patient’s haemodynamic profile may help in better selection and dynamic titration of the therapeutic interventions for CS. Observational studies on heterogenous populations with circulatory shock showed that in up to 60% of cases, invasive haemodynamic assessment led to modification of the therapeutic intervention compared to clinical evaluation alone.54 Similarly, as described above, in the PAC group of the ESCAPE trial in ADHF patients, higher rates of vasodilator therapy were reported, resulting in lower filling pressures at discharge.22
The ultimate treatment goal in patients with CS should be to restore end-organ perfusion without exacerbating the vicious circle of increased myocardial oxygen demand and ischaemia. Unfortunately, because of their intrinsic mechanism of action, neither vasopressors nor inotropes alone can ensure this. Detrimental effects on survival have been shown if recovery is not reached soon after aetiological therapy has been established and higher doses and numbers of drugs are needed to maintain perfusion.55 In this context, there seems to be a pathophysiological rationale for the use of MCS devices to unload the heart and maintain end-organ perfusion in CS. Experimental and pioneering human studies have shown the safety and the potential benefit of a ‘first unload’ strategy in the setting of AMI-CS.
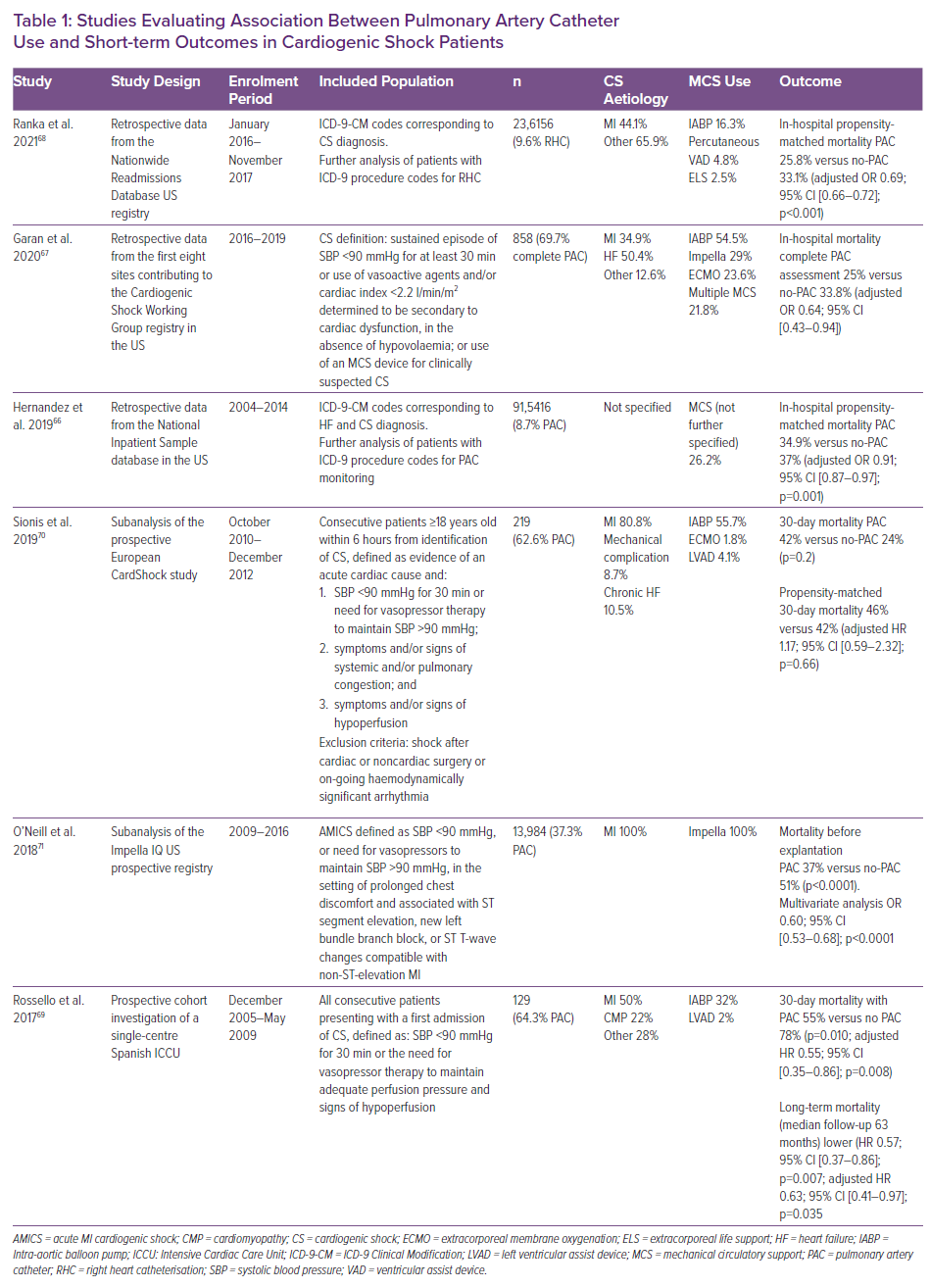
The adoption of timely mechanical unloading may reduce myocardial oxygen consumption and the infarct area, increasing the opportunity for recovery.56,57 In this scenario, the directly and indirectly derived haemodynamic parameters from PAC may finely identify the presence of isolated left-, right- or biventricular failure to define the type of MCS needed.49 In particular, a value of cardiac power output <0.6 W and a PAPi <1 are used in many recent shock management flowcharts as predictors of left ventricular and right ventricular failure, respectively.11,12
Through close monitoring of lung function data and according to the presence and degree of respiratory failure, the clinician may decide if an isolated pump or a combined pump and oxygenator support is needed.58 Notably, multiparametric evaluation including PAC assessment must be approached in a dynamic and prospective way. After the initial profiling, frequent prospective re-evaluation of the aforementioned parameters will allow the identification of patients who are responding or those who are failing and needing an up-titration or palliation strategy. Likewise, considering the well-known risk of time-dependent complications, the duration of MCS should be long enough to achieve effective myocardial recovery or stabilisation toward long term replacement therapies, but adequately short to limit the unwanted consequences of these devices.6,9,59 Continuous monitoring of how the filling pressure and native CO modify while reducing MCS flow during a weaning trial may help determine the timing of safe removal. Adequate device selection in the right patient and timely removal may be the keys to the expected prognostic benefits of MCS in CS patients.
Assessing Candidacy for Long-term Replacement Therapies or Palliation Strategies in Non-responders
Patients who cannot be permanently weaned from their device and/or inotrope or vasopressor therapies must be considered for a long-term heart-replacement strategy, i.e. heart transplantation or left ventricular assist device (LVAD) implantation.
According to the International Society of Heart and Lung Transplantation and the recently published European Society of Cardiology Heart Failure guidelines, RHC maintains a Class I indication as a mandatory screening tool during candidacy assessment.60,61 Evaluation of the presence of pulmonary hypertension (PH) and its severity and reversibility are mandatory before heart transplantation listing. In cases of severe and irreversible PH, the implantation of a LVAD as a bridge to candidacy – or even as a destination therapy – can be considered. Moreover, evaluation of the degree of RV dysfunction through well-validated predictors of post implantation RV failure, such as a reduced PAPi or an increased RAP-to-PCWP ratio, are relevant parameters to be considered before implantation.62 Finally, in cases of irreversible end-organ damage, advanced sarcopenia, lack of a caregiver support or other contraindications for both these rescue strategies, palliation must be considered and undertaken, with patients and their families adequately supported in the end-of-life period.63
Prognostic Implications of Swan-Ganz Catheter Monitoring in Cardiogenic Shock
When evaluating studies investigating the potential prognostic impact of PAC in CS it is important to stress the concept that the presence of a catheter in the pulmonary artery per se does not improve prognosis. Derived haemodynamic data need to be accompanied by appropriate clinical responses to determine the effect on the patient’s clinical course.
No monitoring device can improve patient-centred outcomes unless it is coupled with treatment that itself improves outcomes. This is especially true in the extremely heterogeneous and unstable setting of CS. The lack of uniform practice, together with the absence of RCTs, makes it difficult to draw definite conclusions from previous works. Therefore, some researchers have called for future studies testing standardised protocols to effectively assess how PAC influences in-hospital therapeutic interventions and outcomes.64
While acknowledging these important limitations, it is noteworthy that the majority of previous studies focusing on PAC in HF report better results in the most severe subgroups of patients presenting with hypotension or shock.19 In particular, in a sub-analysis from the historical GUSTO IIb and III trials in over 26,000 ACS patients, those who underwent PAC insertion (2.8%) experienced higher 30-day mortality, with the exception of those presenting in shock for whom the outcome was neutral.65
Similar results were reported in the biggest registry available to date from National Inpatient Sample database.66 In this 10-year retrospective analysis of more than 9 million patients with HF, PAC use was associated with significantly higher mortality (9.9% versus 3.3%) and in-hospital cardiac arrest (2.7% versus 0.6%), although declining with time.66 However, paradoxically, the same study showed that the use of PAC in the CS sub-group correlated with a reduction in both outcomes (9% and 23% decreases, respectively), which was confirmed in the propensity-matched analysis. The authors reported that since 2007 the mortality trend for CS with and without PAC has separated and speculated that this may be in part because of advances of HF therapy and the adoption of MCS where invasive monitoring was largely used.
Several observational studies focusing on the CS population have been published in recent years, with all but one of them showing a protective association between PAC use and short-term mortality after adjusted analysis.67–71 An overview of these studies is reported in Table 1. The Cardiogenic Shock Working Group performed a 3-year retrospective analysis on over 1,400 CS patients from eight tertiary care institutions who had undergone a complete (42%) or a partial (40%) PAC assessment, or no invasive evaluation at all (18%) during the index hospitalisation.67 The main cause for CS in the cohort was decompensation of HF followed by AMI-CS. The complete PAC assessment group had the lowest in-hospital mortality compared to the other groups across all SCAI stages. This result was more pronounced for more advanced stages (stage D and E) and remained significant after adjustment for comorbidities, cause of shock and PAC usage per site.
Ranka et al. recently reported a retrospective evaluation of the Nationwide Readmissions Database (NRD), selecting more than 25,000 patients who had received RHC among the total CS population (9.6%). This subgroup experienced a 31% reduction in adjusted in-hospital mortality and a 17% reduction in adjusted 30-day rehospitalisation, while being six times more likely to receive invasive advanced treatment for HF during rehospitalisation compared to the non-RHC group.68
A smaller, single-centre prospective study by Rossello et al. confirmed that CS patients receiving PAC monitoring (64% of the total) experienced 45% lower adjusted 30-day mortality and 37% lower adjusted long-term mortality.69 The authors underlined that the benefit was only significant in the non-ACS group in their subgroup analysis, but the small sample size clearly limits any reliable interpretation on this point. Moreover, in the previously cited largest NRD cohort, a similar benefit from invasive pulmonary artery monitoring was noted irrespective of the aetiology.68
Another focused analysis of 219 CS patients in the prospective, multicentre European CardShock study showed that only 37.4% of patients had PAC monitoring. They had worse baseline profiles and were treated more aggressively and more frequently with MCS. The authors did not find any survival impact of the PAC implementation in a small propensity-matched cohort of two sets of 50 matched patients.70
Finally, a larger cohort of AMI-CS patients treated with the Impella device found a benefit in terms of survival to device removal for those who had received invasive monitoring during support.71 As previously underlined, the context of CS patients needing MCS seems to be an interesting window of opportunity for Swan-Ganz monitoring to improve ability in device selection, upgrading and timely weaning. It may reduce the risk of related complications and lead to the much-awaited survival improvement.
Association of PAC Monitoring and Therapeutic Interventions in Cardiogenic Shock
Patients with CS undergoing invasive monitoring are usually those receiving more aggressive therapeutic approaches because of their worse clinical condition as demonstrated by baseline characteristics. Few studies in this setting reported details on the rate and type of inotrope/vasopressor used in the PAC and no-PAC groups, confirming a higher adoption rate in the first group.69,70 In terms of MCS used, all of them but one showed a higher MCS implantation rate within the cohort monitored with Swan-Ganz catheter.56
Safety of Swan-Ganz Monitoring
Invasive monitoring with PAC insertion is associated with a small incidence of complications. Previous studies suggested that the most frequent complications are related to the site of catheter insertion (up to 3.6%) and strictly depend on the specific centre’s experience.72 Rarely, severe complications such as heart block (0.3–3.8%) and pulmonary artery rupture (<1 case per 1,000 insertions) may occur.72 Among previously cited studies focusing on the critical CS setting, only three describe data on the incidence of complications related to use of the Swan-Ganz catheter.66,69,70 Overall the incidence ranged between 5% and 10%, but few details on the type and clinical impact were provided.
Conclusion
CS is still burdened by very high short-term mortality despite therapeutic and technological improvements in recent years. The key for improving prognosis probably relies on an integrated approach with timely diagnosis and phenotyping, a shock team and a management protocol. The Swan-Ganz catheter, whose use has clearly dropped in the last 50 years, is being rediscovered as a valuable tool and may play a role in steps from diagnosis to weaning from MCS. Few studies on its prognostic implications in this setting have been published to date. Considering the intrinsic limitations of observational studies, further prospective evidence is needed to better clarify whether the theoretical usefulness of this diagnostic tool will help in achieving the – as yet unmet – goal of improving survival in this disease.